Summer Undergraduate Research Scholars
We provide members with both technical training in exciting new research areas and the professional development training they need to thrive in 21st century careers in industry, national laboratories, academia and entrepreneurial activities.
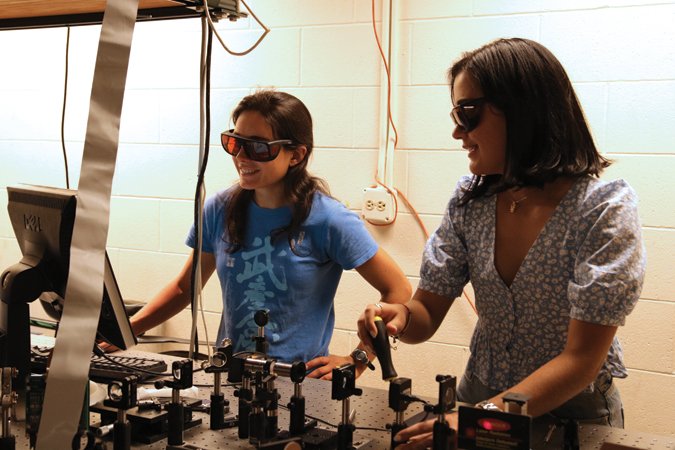
Deadline for Application
EXTENDED DEADLINE:
February 7, 2025
Selection decisions will be sent starting in early March, and the selection process will continue until all positions are filled.
ATTENTION APPLICANTS
If you submitted your application between 1/16/25 and 1/29/25, or did not receive a confirmation email, please resubmit your application.
The application portal will remain open until Friday, February 7, midnight PT.
STROBE is an NSF Science and Technology Center coordinating six institutions across the US to build the microscopes of tomorrow. Our research includes visible, x-ray, nano-probe, and electron microscopy, and has applications across materials science, basic physics and chemistry, and biological systems. We have research opportunities at the University of Colorado at Boulder, the University of California at Los Angeles, the University of California at Berkeley, Fort Lewis College, Florida International University, and the University of California at Irvine (see below for specific research descriptions at each site).
All programs include a $5,000–6,000 stipend and additional funding may be available for housing/travel for those who need it.
Program dates vary but are typically 8–10 weeks. We can accommodate students from both semester and quarter system schools. The program includes research in a mentored lab experience, supporting group and social activities, community networking, and academic, science, and career development opportunities.
You can find information and tips for preparing your application on our FAQs and Application Tips + Templates pages.
Please direct questions to STROBE’s Director of Education, Dr. Ellen Keister.
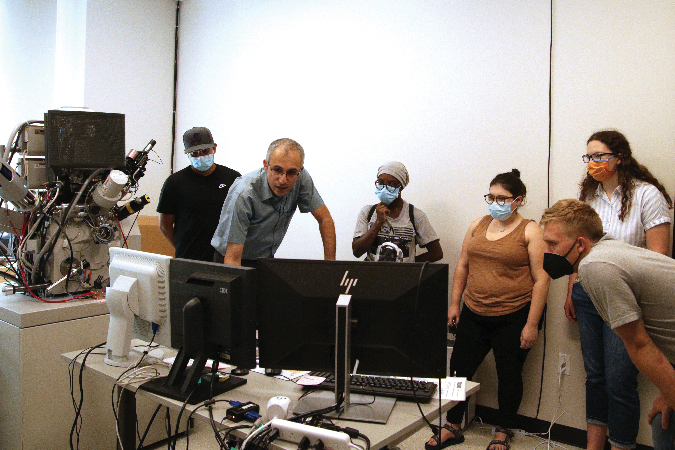
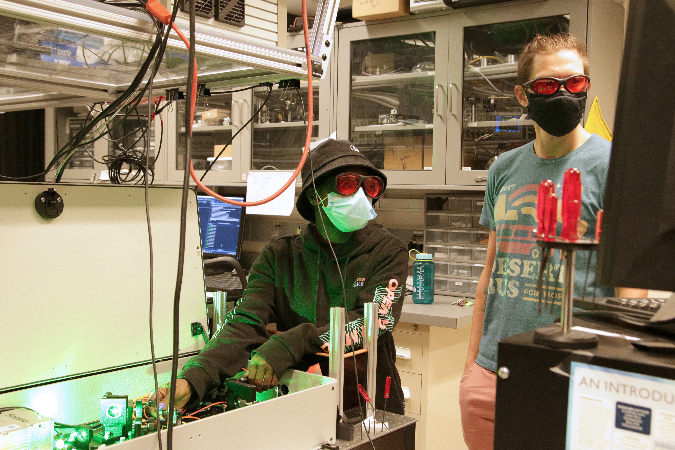
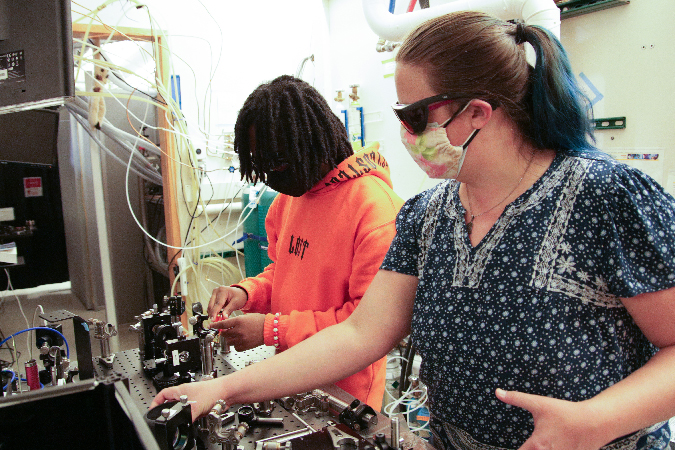
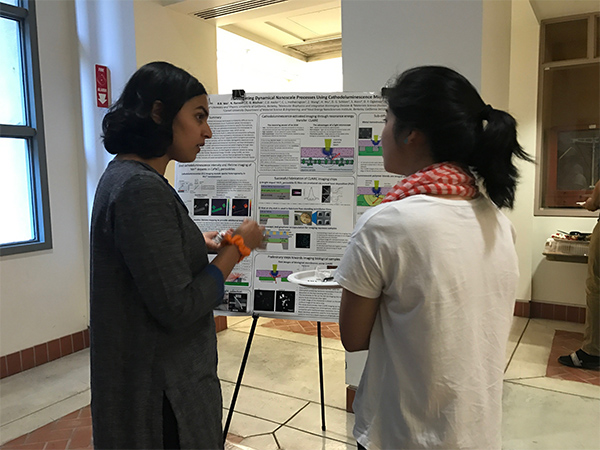
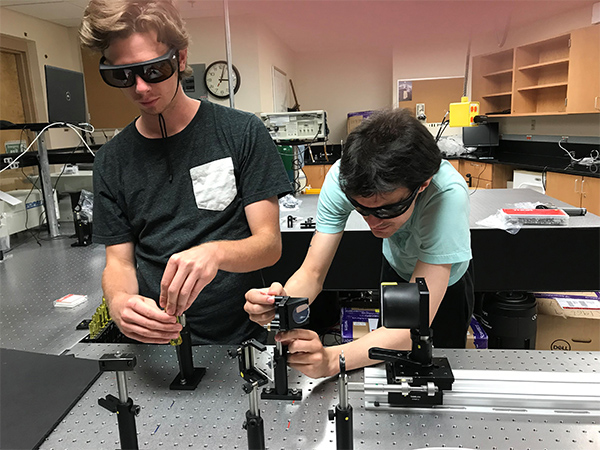
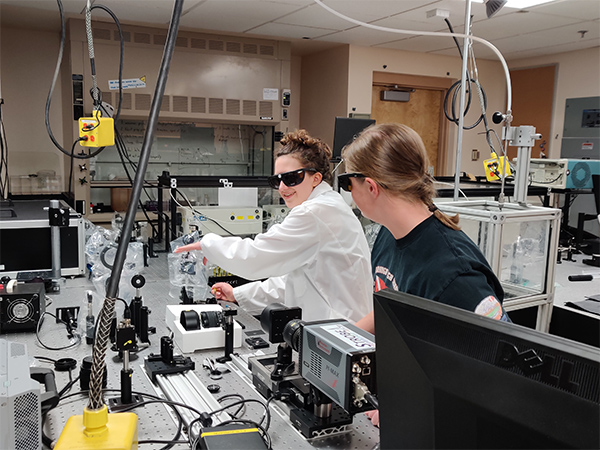
STROBE Labs Participating in the SURS Program
University of California, Los Angeles
The Kogar lab uses intense light pulses to discover, study and control various states of matter including charge density waves, superconductors and other exotic phases. At a high level, the lab seeks to answer the following questions pertaining to this topic: (1) What kinds of phases of matter exist away from thermal equilibrium? (2) Can the different degrees of freedom of light, i.e. the polarization, wavelength, orbital angular momentum, etc., be used to control various phases? (3) Can light be used to create and manipulate topological defects in ordered phases? To answer these questions, the group uses ultrafast electron diffraction and time-resolved second harmonic generation.
The Kogar lab is also working on developing spectroscopic tools to quantitatively measure the Coulomb energy change across phase transitions in order to place various constraints on how solids save energy. This is particularly pertinent in the field of unconventional superconductivity, where there is still much debate about where the energy is saved as a material undergoes a change from its “normal” to superconducting phase. As part of the ultrafast electron diffraction beamline, the lab is designing transmission electron energy loss spectroscopy instrumentation to perform such experiments.
Suggested Skills: Some knowledge of Python and/or Matlab is preferred.
Suggested Skills: Upper Division E&M and/or Classical Mechanics preferred. The project will be mostly theory/computation, as it will be related to the design of electron optics for a variable magnification microscope, but there could be practical aspects of it (for example measuring magnets). Will mostly use commercial software that there is prior knowledge of programming needed. Some knowledge of Matlab to interpret and postprocess data might be useful.
The Regan group includes interests that are both fundamental and applied. Their long-term goals include a better understanding of the overlap between thermodynamics and quantum mechanics, and the construction of a model system for the investigation of clean energy harvesting. Our areas of expertise include carbon nanotubes, graphene, nanofabrication and in situ transmission electron microscopy.
The Zhou lab investigates the 3D structural studies of biological complexes using cryo-electron microscopy (cryoEM) and cryo-electron tomography (cryoET). These emerging methods are particularly suitable for structure determination of large molecular complexes, viruses, cellular machineries and bacterial cells. Recent efforts have focused on developing and applying advanced cryoEM and cryoET techniques to visualize the dynamic processes of microbial infections and to decipher the mechanisms of fundamental biological processes.
University of California, Berkeley
Our research group designs and synthesizes new atomically thin, precisely tailored two-dimensional (2D) materials in which the collective behavior of electrons can be studied and exquisitely controlled. We leverage these materials to uncover the principles that underlie efficient manipulation of electron transport within solids—the basis for novel ultralow-power electronic devices—and across solid–liquid interfaces—enabling the next-generation of fuel cells and electrolyzers for renewable energy conversion and storage. To achieve our goals, we leverage solid-state and solution-phase methodologies, as well as chemical and electrochemical deposition techniques for materials synthesis. We use state-of-the-art micromanipulation and nanofabrication tools for the preparation of mesoscopic structures, and employ a range of optical spectroscopy, scanning-electrochemical, electron microscopy, and low-temperature quantum magnetotransport probes to measure the (electro)chemical and physical properties at individual devices.
Currently open research projects include mechanism-guided electrocatalyst discovery for fuel-forming and fuel-consuming reactions in electrolyzers and fuel cells; ion insertion and transport reactions of two-dimensional heterointerfaces for energy storage and to create novel quantum materials; and the electro-chemical control of light–matter interactions and topological phases in 2D semiconductors and semimetals.
Suggested Skills: any experience with programming (Python, matlab or C/C++), image manipulation or low power lasers.
Suggested Skills: A background in Matlab/Python and image processing is desirable.
Suggested Skills: Familiarity with MATLAB and Python.
Our research is inherently interdisciplinary, drawing from expertise in optical physics, signal processing and computer science, with broad applications in bioimaging, defense, physical science and industrial inspection. We work with optical microscopes, consumer cameras and X-ray imaging systems, and we use algorithms of nonlinear non-convex optimization, compressed sensing and machine learning.
Prof. Xu and his research group develop new experimental tools to interrogate biological, chemical, and materials systems at the nanoscale with extraordinary resolution, sensitivity, and functionality. To achieve this, the group takes an interdisciplinary, multidimensional approach that integrates advanced microscopy, spectroscopy, cell biology, and nanotechnology. Recent developments include spectrally resolved and functional super-resolution microscopy, ultrahigh-throughput single-molecule spectroscopy, interference-based microscopy for 2D materials, and uncovering the nanoscale ultrastructure of the membrane cytoskeleton and intracellular vesicles.
University of Colorado Boulder
Our group is particularly interested in whether fluorescent proteins (FPs) can be engineered to have photophysical properties, such as brightness, that are superior to those of conventional organic fluorophores, and if so, what are the structural and dynamical features of FPs that enable these properties? We use a combination of random and targeted mutagenesis to create and assess new red/far-red FPs with improved spectral and cellular properties. We have designed and operated several novel microfluidic systems for screening these large libraries of mutants. For example, microdroplets containing 1–3 bacterial cells, each expressing a unique protein variant, can be individually excited by a chosen laser wavelength, their emission assessed via a customized detection system, and the droplets/cells of interest collected through a gated channel. Thus, we can enhance for a cell population carrying FPs with particular qualities.
Suggested Skills: Motivation to succeed.
Suggested Skills: Motivation to succeed.
Fort Lewis College
Dr. Lamb’s research focuses on following the flow of energy through photocatalytic systems—particularly visible-light absorbing organic photoredox catalysts–which are critical to the kinds of sustainable and green chemistries that could greatly offset energetic expenditure in the bulk chemical industry (1/9th of our country’s energetic expenditure). However, this technology is in its infancy and optimizing the methodology is necessary to achieve efficient processes. Student researchers will investigate both the photophysical properties of these catalysts as well as the photochemical reactions that they conduct with model substrates relevant to the production of polymeric materials. Much of the research will include spectroscopic and electrochemical measurements and analysis.
The current mission of the Embedded Systems lab at Fort Lewis College is to detect low-concentrated microorganisms, particles, organic matters from a large volume of liquid using optical-electro techniques (fluorescence and Raman Spectroscopy). The targets can be single-molecule DNA, bacteria, fluorescence particles, proteins, or Amino acid. The detection techniques are Droplet Digital PCR, rtPCR, Raman Spectroscopy, and High-Speed Imaging. The lab is currently funded by NSF, EPA, and the Department of Education.
The lab is equipped with modern bench-top electronic testing instruments, a Photron MimiUX 32 GB high-speed camera (>5000 fps), an AmScope fluorescence microscope, various microcontroller and embedded system kits, FPGA demo boards, an integrated circuit wire bonder, Cadence Virtuoso IC design environment, a GPU work station (5000 GPU cores), programmable LED lasers (200 mW), and microfluidic device fabrication facilities.
Florida International University
University of California Irvine
The work done in the Ultrafast Laser Plasma Interactions lab focuses on generating compact radiation sources in high intensity laser matter interactions. Laser pulses with durations of femtoseconds can achieve extreme intensities which can instantly ionize matter into plasma and drive electrons relativistic in a single optical cycle. This highly complex and nonlinear regime is capable of generating particle beams of electrons and light ions, as well as coherent x-ray sources. We study these interactions both through experiments in vacuum chambers as well as numerically with high performance computing. SURS scholars have performed projects on Laser Wakefield acceleration, high harmonic generation, laser mode shaping, and coherent diffractive imaging.
SURS Past Participants
Pietro Musumeci
(2019) Amir Amhaz, “Designing and Simulating RF Compression Cavity”
(2017) Aline Tomasian, “Permanent Magnet Quadrupoles for Relativistic Electron Imaging”
John Miao
(2019) Jena Shields, “Cryogenic Electron Tomography Reconstruction”
(2018) Stavrini Tsagari, “Revealing the history of our solar system through X-ray spectroscopy”
Jose Rodriguez
(2019) Hannah Hoffmann, “Crystallizing amyloidogenic peptide segments from the amyloid protein LECT2”
(2018) Ayesha Hamid, “Structural Analysis of Enantioselective Peptide Nano-assemblies by Micro Electron Diffraction”
(2018) Ronquiajah Bowman, “Curing Disease by Understanding the Structure of Brain Prions”
Hong Zhou
(2019) Julia Greenbaum, “Particle-Picking Diffocins, Bacteriocins of Clostridium Difficile”
(2018) Phebe Ozirsky, “Cryo Electron Microscopy for Structure Determination of Spliceosomes”
Roger Falcone
Naomi Ginsberg
(2018) Namrata Ramesh, “Synthesizing Various Shapes of Mn Doped Perovskite”
Andrew Minor
(2019) Jay Aindow, “3D Hardness Mapping”
(2018) Hillal Ibiyemi, “Automated Feedback Control for Alignment of Laser Beams in Microscopes”
(2018) Brian Chen, “Correlated nanobeam diffraction and tip-enhanced Raman spectroscopy measurements”
Colin Ophus
Mary Scott
Laura Waller
(2020) Eric Li
(2019) Matthew Wells, “Characterizing 3D Fluid Flow Around V. Convallaria Via Inline Holography”
(2018) Lena Blackmon, “Seeing the Micro-Invisible: Phase Imaging via Off Axis Holography”
(2017) Camille Biscarrat
(2017) Shreyas Parthasarathy, “DiffuserCam: Diffuser-based Lensless Imaging”
Ke Xu
Margaret Murnane and Henry Kapteyn
(2020) Matthew Jacobs, “High-Resolution, Quantitative, Complex Beam Polarimeter”
(2020) Hannah Hoffman, “Simulating a Grism Stretcher using Zemax”
(2019) Baldwin Varner, “Uncovering the hidden charge density wave phase in TaSe2”
(2019) Matthew Jacobs, “Characterizing Fibers for Laser-like X-Ray Generation”
(2019) Allison Liu, “A Comprehensive Characterization of Orbital Angular Momentum Beams using Gerchberg-Saxton”
(2018) Hannah Hoffmann, “Optimizing a lensless microscope using ptychographic coherent diffractive imaging”
(2018) Juan Boza, “Construction of a Hybrid Fourier Microscope Incorporating Visible Light and X-rays”
(2018) Paul Adelgren, “Imaging Magnetic Topological Features with Magneto-Optical Kerr Effect Microscopy”
(2018) Christian Montes, “The Extension of Imaging by Integrated Stitched Spectrograms”
Heather Lewandowski
Markus Raschke
(2019) Caleb Wexler, “Correlative Mapping of Chemical Heterogeneity”
(2018) Andrew Voitiv, “Ti:Sapphire laser construction for characterization of thermo-plasmonic nanotips”
(2018) Diana Rossell-Eddy, “Development of an Ultraviolet- Visible Absorption and Photoluminescence Spectrometer for use in the Characterization of FAMAC Perovskite and other energy-related materials”
Rafael Piestun
(2018) Gwendalynn Roebke, “”Propagation of Light through Turbid Media: Observing and drawing conclusions about the composition and behavior of tissue mimicking materials as based on their diffusive properties”
Noah Finkelstein
David Blake
Jeff Jessing
(2020) Cooper Wiens, “Lung-on-a-Chip: Porous Silicon Membranes & Cell Co-Culture”
(2020) Jessica Fiala, “Lung-on-a-Chip: Fluidic Modeling of the System”
(2020) Javionn Ramsey, “Porous Silicon Rocket”
(2019) Cooper Price Wiens, “Lung-on-a-Chip/Live-Cell Imaging Platform: Overview”
(2019) Madeline Stadler, “Lung-on-a-Chip: Thin Silicon Through-Wafer Anodization Process Development”
(2019) Avery Killifer, “Lung-on-a-Chip: Cell Co-Culture Methodology Development”
(2019) Lily Vonesh, “Lung-on-a-Chip: Bulk Silicon Thinning Process Development”
(2019) Elizabeth Blackwater, “Porous Silicon Rockets”
(2018) Alexander J. (AJ) Biffl
(2018) Nate Curmano, “Developing Sprays for Leading Edge Imaging Diagnostics”
Yiyan Li
(2020) Denzel Farmer, “Bacteria Identification with Raman Spectroscopy: The Network”
(2020) Nic Theobald, “Bacteria Identification with Raman Spectroscopy: Machine Learning and Neural Networks”
(2020) Keenan Harvey, “Bacteria Identification using Raman Spectroscopy: Surface Enhanced Raman Scattering”
(2020) Kaitlyn Kukula, “Bacterial Identification with Raman Spectroscopy: Data and Model Visualization”
Megan Paciaroni
(2019) Kat Detmer, “Particle Image Velocimetry via Optical Time-of-Flight Sectioning (PIVOTS)”
(2019) Jessica Fiala
(2019) Nicole Wiley
(2018) Jodi James, “Quick-shot Spray! Backscattering Image of Sprays”
(2018) Nathan Keith, “Image Processing For Particle Sprays”
(2018) Michael Wolfersperger, “Two-Photon Laser Induced Fluorescence Imaging with an Optical Kerr Effect Gate”
(2017) Hannah Hoffman, “Interferometry, Holography, and Microscopy”
(2017) Paul Adelgren
Jessica Ramella-Roman
(2019) Romin Patel, “Mueller Matrix Imaging via Polarized Microscopy”
(2019) Michael Ricardo, “Mueller Matrix Imaging via Polarized Microscopy”
(2018) Michelle Foreman, “Non-destructive Microscopic Visualization of Nerve Fascicles”
(2018) Maily Hernandez, “Non-destructive Microscopic Visualization of Nerve Fascicles”
Jin He
(2019) Alex Rodriguez, “Custom Incubator for Microscopy Imaging”
(2019) Antonio Martinez, “MATLAB interface development for 3-Axis Optical Stage with Piezos and Scientific Camera for an Epi-Illumination Fluorescence Microscope”
Franklin Dollar
(2019) Mirella Soto, “High Repetition Rate Targetry for Laser Driven Ion Accelerators”
(2018) Yasmeen Musthafa, “Coherent Diffractive Imaging: Phase Retrieval and Ptychography”
(2018) Tina Tran, “Coherent Diffractive Imaging: Significance”
(2018) Alfred Macias, “Variables Affecting Student Performance in Introductory Physics Classes”
(2018) Danny Attiyah, “Creating Custom Wavefronts for Terawatt Laser Systems”
(2018) Amanda Bell, “Coherent Diffractive Imaging: Experimental Setup and Noise Reduction”
(2017) Edgar Ibarra
(2017) Cheyenne Nelson, “Spectral Tuning of High Harmonic Generation (HHG)”